Personalized Dog Treatments Tailoring Treatments for Your Canine Companion
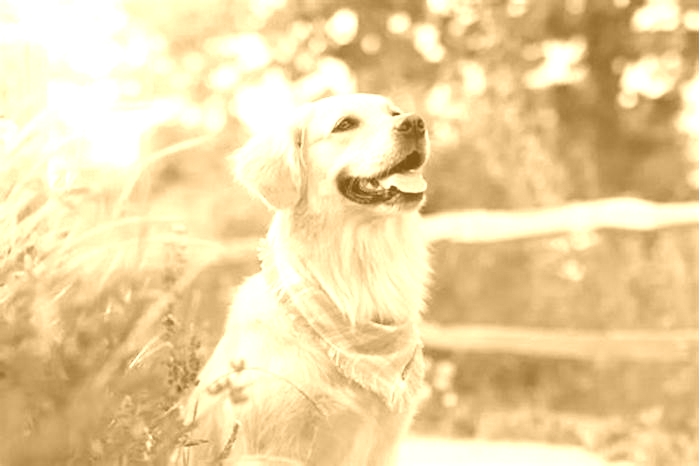
The growing role of precision and personalized medicine for cancer treatment
Once biological data have been acquired and stored, they must be analyzed, with the goal of identifying biomarkers, mutations, or pathways relevant to disease or treatment outcome. The field of systems biology aids in these efforts by analyzing data from preclinical and clinical studies. Statistical and modeling techniques are used to identify and assess mechanistic relationships within biochemical systems. This analysis is used to develop predictive tools that replicate biological systems in order to characterize their behavior and response in the context of disease and drug development. This is particularly relevant to forthcoming cancer treatments, as approximately 73% of oncology drugs in development are personalized medicines9. PPM therapies that are currently being developed include cancer vaccines, mAbs, and CAR T-cells. Organoids are being used as in vitro models to understand tumor heterogeneity and the variability of patient response to cancer treatments. As these PPM products and services emerge, it is pertinent that companies are aware of the evolving regulatory landscape for the PPM field and continuously reference updated guidance documents.
Linking omics data to treatment
A major challenge in PPM lies in establishing the relationship between biological data, disease, and clinical translation: how can we interpret the data collected to make meaningful medical decisions? Big Data, in reference to the medical industry, refers to the greater collection of medical data across thousands of patients, involving the tracking of various medical indicators and biomarkers (primarily clinical and omics data). High-throughput data collection enables researchers to screen tissues for thousands of molecular targets, effectively capturing the response of a complex system over time. Within the field of systems biology, reconciliation of these omics components enables the construction of predictive models of human physiology used in experimental design and clinical trial development6063.
In order to correlate observations with biological events and phenotypes, systems biologists and bioinformatic scientists employ techniques to identify statistically significant trends64. These include multivariate decomposition techniques, predictive modeling and optimization techniques, and other statistics-based tools. Statistically interpreting trends from Big Data is a discipline unto itself and is necessary for predictive modeling and clinical decision support65.
It is important to remember that Big Data is a network of information that is both useful and deafening analysts of which suffer the burdens of missing values, curse of dimensionality, and bias control65. Big Data is not an easy-to-use information source from which trends connecting diseases to patient characteristics can be simply identified. Instead, Big Data is a multidimensional network containing medical information from thousands of patients, all of whom are influenced by different environments, have unique genomes and epigenomes, and who are analyzed by different physicians prone to unique biases and varied techniques. Furthermore, patient screening, sample collection and analysis, and even physical measurements are all subject to bias. All of these considerations contribute to the complexity of the PPM field.
Omics tests and clinical trials
Omics data is essential to the development of targeted therapies as well as patient stratification, notably within preclinical experiments and clinical trials. Le Tourneau et al. reviews specifications for establishing PPM clinical trials those that select patients likely to respond to the experimental treatment, as determined by molecular profiling of tumors66. This field of study, called pharmacogenomics, uses experimental and quantitative sciences to analyze the influence of genomics on drug effects67. Diseases and drug effects are conventionally correlated to macro data, such as age, weight, and gender. Improvements in high-throughput screening technology and increasing reliance on computational tools have enabled the development of the pharmacogenomics field, which correlates drug effects with omics data while revealing gaps in which drug targeting can be made more specifi c67. Such pharmacogenomic analysis can ultimately identify patient populations likely to be responsive to targeted therapies, which is a primary goal of PPM.
To get from omics data to patient stratification in clinical trials, predictive computational models must be used. Here, each molecular target from the omics measurements is a variable in a complex system that represents the tissue6870. Statistical techniques are applied in order to segregate noise from usable information and ultimately reveal physiological trends based off of key molecular markers. The resulting in silico model is further modified by data-driven investigations whose results are analyzed and fitted to mathematical models. Validation of these models is accomplished with additional training data. Once verified, the model is predictive and can be used in further experimental design or clinical trial guidance ()60.
Predictive model development from large-scale omics data. An overview of the process for development of predictive models. Turning gigabytes of patient data into relevant clinical information requires a Big Data approach specifically, predictive algorithms that are refined and validated with results from data-driven investigations, including traditional animal model studies and clinical trials. Adapted by permission from [RightsLink Permissions Springer]: [Springer Nature] [NATURE BIOTECHNOLOGY] Butcher et al.60, [COPYRIGHT] (2004).
The combination of omics assays and a specific computational model (omics predictor) is defined as an omics test63. There are two types of omics tests: prognostic test, which predicts a clinical outcome in the form of a measurement; and a therapy guiding test, which identifies subgroups of patients that are unique in their response to a particular therapy63. Notably within the realm of cancer research, omics tests are applied in identifying and validating biomarkers for disease indications61. Establishing the validity of the biomarker for a disease indication requires validation using an omics test, and this omics test (sample preparation, performing the sequencing assay, computational pipeline for assessing the sequence read) also requires validation61. Clinical viability and utility of the biomarker and omics test must be established, meaning that the use of this biomarker should result in the end-point of progression-free survival61,71.
Clinical trials examining the efficacy and utility of validating biomarkers with omics tests are not yet commonly successful (see the National Academy of Sciences report Committee on the review of omics-based tests for predicting patient outcomes in clinical trials for an extensive review71). In short, putative biomarker identification is expedited with omics analyses; however, establishing clinical validity and clinical utility is more difficult. A contemporary primary research focus is the effort to establish safe and effective use of omics tests in clinical trials6163. More specifically, the development of novel and robust statistical analysis methods must undergo the same rigorous development as bioassays63. To enable this, the Institute of Medicine established guidelines for the use of omics analyses in clinical trials in 2013, which the U.S. National Cancer Institute (NCI) adheres to when reviewing proposals for studies involving omics tests61,62. In addition to providing recommendations for application of omics-based tests in clinical trials, these guidelines require that agencies receive FDA approval for all sequencing assays and their associated analysis software tools as potential investigational devices [and provide] public availability and transparency of raw data as a means to enable the external validation of omics-based trials61,62. McShane et al. of the NCI also indicates that as researcher teams with greater variety of expertise (laboratory, computational, bioinformatics, and clinical) develop, omics tests will become more robust63. These institutes and contemporary researchers expect an increase in the number of successful clinical trials incorporating omics tests as expertise expands and rigor improves.
Clinical outlook for PPM cancer products
Advancements in omics technologies have led to drug discovery approaches for a variety of PPM cancer products72. Detection methods for circulating tumor cells (CTCs) and DNA are promising not only for early diagnosis, but also for individualized patient risk monitoring and identification of effective personalized treatments. Another approach has focused on recapitulating individual tumors in vitro, in order to determine the safest and most effective treatment before administration to a patient. Several other therapies under development harness the unique power and specificity of the immune system to combat cancers. Over a century of work has focused on this and has evolved into a distinct discipline called immunoengineering. The ultimate goal of this field is to tailor an increasingly specific and potent immune response, which can result in a powerful, effective, and personalized cancer treatment73.
CTCs and DNA for early cancer detection
Two types of oncological biomarkers, CTCs and circulating tumor DNA (ctDNA), have emerged as the face of the liquid biopsy techniques focused on noninvasive cancer diagnostics. Research supporting the notion that tumors shed both types of biomarkers into the bloodstream early on in cancer progression has meant that much focus has been placed on their applications for early detection and screening7476. As research continues and technology improves, CTCs and ctDNA are also likely to prove useful in risk stratification, disease monitoring, and personalized treatment selection.
The biggest challenge in implementing CTC detection techniques is the rarity of these biomarkers: estimates place CTC frequency at one cell per 106107 leukocytes77. Thus, CTC detection techniques require some form of sample enrichment or isolation step, such as immunoaffinity/ antibody targeting of cell surface markers, size exclusion methods, or separation on the basis of electrical properties. These sample preparation steps are not without their own issues: CTC viability can be negatively affected by these processes, the heterogeneity inherent to CTCs means not all cells may be detected, and the lack of standardized protocols has resulted in significant variability in results between techniques, operators, and laboratories77. However, once captured, CTCs can provide a wealth of omics information through single-cell next-generation sequencing (NGS), migration assays, RNA-Seq, and EPISPOT immunoassays7779. Perhaps the most intriguing potential applications of CTCs are personalized functional assays using patient CTC xenografts in mice or in vitro cultures80. Such an assay has already been used to assess the efficacy of drugs in prostate cancer patients, with assay results corresponding well with patient drug resistance status81.
Unlike CTCs, ctDNA does not require specialized sample preparation steps for detection and can often be detected in samples in which CTCs are absent79,82. ctDNA is likely released by apoptotic or necrotic cells within a tumor, or by the destruction of CTCs via apoptosis, the immune system, or anoikis77,82,83. Like CTCs, increased levels of ctDNA are generally associated with later stage disease or disease recurrence after treatment. The two primary types of information that can be gleaned from ctDNA are mutation status and methylation status, though a limited degree of copy number variation analysis may also be possible. ctDNA mutations can be assessed by a variety of techniques including allele-specific polymerase chain reaction (PCR), digital PCR, and tagged-amplicon deep sequencing (TAm-Seq)82. ctDNA mutation status could play a key role in monitoring disease progression during treatment and checking for the presence of drug-resistant subclones74,76. Methylation status is typically assessed with methylation specific PCR and can be used to reliably distinguish ctDNA from nontumor derived cell-free DNA. Major challenges that ctDNA diagnostics face include lack of standardization (such as how many mutations constitute a positive result when used for screening), potentially confounding mutations due to clonal expansion of benign cells, and difficulty in establishing personalized assays for the general population, that is, those without an established history or risk of cancer76.
Organoids
One approach currently under development for personalized treatment of cancers is patient-derived tumor organoids, which serve as in vitro tumor models and predictors of drug responses84. Traditional approaches to cancer research and therapies involve the use of in vitro cancer cell lines, patient-derived xenografts, and 3D culture models. These are limited by their inability to accurately correlate an individual tumors response to a treatment due to the diversity and heterogeneity of the tumor microenvironment. Organoids offer a more accurate representation of this dynamic niche and there is evidence that the genomic and functional resemblances between patient-derived tumor organoids and their original specimens can be nearly identical8588. The original success of tumor organoid cultures came from Weeber and colleagues, who successfully reported 90% preservation of somatic mutations and DNA copy number profile between the developed tumor organoids and patient original biopsies. This was achieved across a total of 1,977 cancer-related genes from 14 patients with metastatic colorectal cancer85. Other positive developments in the use of these organoid models were reported by van de Wetering et al.86 The group successfully established a biobank of 20 colorectal carcinoma (CRC) derived tumor organoid cultures. Each culture represented a major CRC mutation subtype that was confirmed by whole-exome sequencing analysis. This allowed for a more accurate detection of genedrug associations for each individualized subtype of CRC. Another promising study conducted an examination of drug sensitivities of tumor-derived organoids against a library of 63 drugs in 232 treatment regimens89. Tumors resected from 14 patients with refractory advanced cancers were propagated in mice and treated. Researchers were successfully able to identify an effective treatment for 12 of the 13 individual patients in the xenograft model. Therefore, 11 of the 12 patients received their prospectively guided treatments, with one patient having died before treatment. This data supports the use of personalized xenograft models for guided treatment platforms. Tumor-derived organoids provide a means for an accurate representation of genedrug association on an individual basis, with the ease-of-use of an in vitro model. Hence, organoids hold immense potential to play significant roles in the development of PPM cancer therapies.
Targeted mAbs for cancer therapy
Out of the many molecular-based techniques (e.g., small molecules, mAbs, and vaccines), mAbs have been very promising for cancer therapeutics due to their low cytotoxicity, high specificity, and scalability9092. mAbs are Y-shaped proteins, produced either synthetically or by B lymphocytes, that have the ability to bind to a specific molecular target. mAbs are one of the fastest growing immunotherapies; there are over 22 FDA approved mAbs-based drugs for oncology.
In contrast to traditional therapies (e.g., surgery, radiotherapy, and/ or chemotherapy), therapies based on mAbs are targeted to specific molecular markers that a particular tumor expresses, and are therefore likely to be more effective. For instance, human epidermal growth factor receptor 2 (HER2) positive breast cancers result in better clinical benefits from HER2-targeted mAbs (e.g., trastuzumab and pertuzumab) than mAbs that target HER2 negative breast cancer markers (e.g., everolimus)93. Additionally, epidermal growth factor receptor (EGFR) mAbs are commonly used for treatments of KRAS wild-type colorectal tumors, but nearly half of treated patients have not shown any clinical benefits94. Interestingly, under some conditions, tumors even continue to mutate and develop primary resistance against the targeted molecule95. Ultimately, the choice of the mAb (or the combination of mAbs) will often be defined by the cancer type, cancer subtype, and overall efficacy and side effects from other clinical and preliminary studies.
Recent advances in NGSTs at the single-cell level have provided researchers with more precise information about novel drug targets. This work has improved mAbs that target specific antigens on cancer cells and resulted in a more personalized approach9699. Mercks pembrolizumab (Keytruda) became the first drug to target a genetic signature (biomarker PD-L1 expressed in 50% of the non-small cell lung cancer) rather than a disease100. In a Phase III clinical trial, treating patients with pembrolizumab, combined with a first line chemotherapy drug, resulted in a 36% higher response rate and lower side effects compared to treating patients with only chemotherapy101. Recently, mAbs in combination with other mAbs or chemotherapy have entered mainstream targeted cancer therapy. In addition to cancer, mAb therapies are also used to treat autoimmune diseases, infection, and hematological diseases. With increasing demand for PPM, current projections reveal that the global mAb therapy market is projected to grow to approximately $1.5 trillion by 2021 and would account for about 20% of biopharmaceutical market share102.
Immune checkpoint inhibitors
A promising advancement in cancer treatment is the development of antibodies capable of blocking coinhibitory immune cell receptors, or immune checkpoints T-cell surface receptors that, when activated by particular ligands, reduce the T-cells cytotoxic immune response. Tumor cells tend to overexpress the ligands that activate these inhibitory receptors, thereby evading the T-cell immune response and proliferating freely103. Though over two dozen different costimulatory receptors have been identified104, two CTLA-4 and programmed cell death 1 (PD-1) have been the focus for antibody-based immune checkpoint blockade (ICB) treatments, and six such drugs have been approved by the FDA105. CTLA-4 was the first identified negative regulator of T-cell activity106; when activated, it delivers inhibitory signals blocking T-cell proliferation and secretion of T-cell maturation agent IL-2107. The CTLA-4 inhibitor ipilimumab became the first FDA-approved ICB drug in 2011 after a clinical trial demonstrated its beneficial impact on survival rates in stage III and IV melanoma patients108. PD-1 was identified as a coinhibitory T-cell receptor in 1999109 and, unlike CTLA-4, represses T-cell activity primarily by promoting T-cell exhaustion110. The first PD-1 targeting ICB drug, nivolumab, was approved by the FDA in 2014, following favorable outcomes compared to chemotherapy in a clinical study administering nivolumab to patients whose melanoma progressed after ipilimumab treatment111. Since then, nivolumab has received FDA approval as a first-line treatment in non-small-cell lung cancer112, renal-cell carcinoma113, urothelial carcinoma114, Hodgkins lymphoma115, and more. A second key anti-PD-1 ICB drug, pembrozilumab, has FDA-approval for similar treatments and also recently became the first anticancer drug to receive site-agnostic approval it is cleared for use on all mismatch-repair deficient solid cancers, regardless of tissue type, with particular biomarkers116. The newest FDA-approved ICB drugs, including atezolizumab117 and durvalumab118, target PD-L1, the PD-1 ligand, thereby providing the same inhibition of PD-1 activation via a different chemical approach. Combinatory approaches involving simultaneous use of both CTLA-4 and PD-1 or PD-L1 inhibitors119, or PD-1 inhibitors with additional T-cell costimulators120, are currently under development with promising preliminary results.
In the context of PPM, effectively using these therapies will require diagnostics to determine the likelihood of a particular patients tumor responding appropriately to the ICB drug. Further investigations into the cellular mechanisms of the immune checkpoint are undergoing, with the aim of identifying biomarkers and other diagnostic features that could predict a patients response to this immunotherapy121. Potential biomarkers for anti-PD-1-based therapies include direct assessment of PD-L1 expression, density of tumor-infiltrating lymphocytes, and quantity of mutation-related neoantigens in tumor cells; effective treatment will likely require using a combination of these and unknown other markers122. The frequency of CD4 T-cells expressing the inducible costimulator (ICOS) marker has been found to be a robust pharmacodynamic biomarker for anti-CTLA-4-based treatment efficacy123. Development of clinical tests using these and other potential markers will enable a personalized immunotherapy approach for a wide variety of solid cancers.
Cancer vaccines
Cancer vaccines, which have long been envisaged as effective tools for cancer immunotherapy, are designed to amplify the tumor-specific T-cell response through active immunization124. Through selection of a suitable antigen target present on tumor cells, a potent and tumor-specific immune response can be induced. Studies have shown that tumor neoantigens, or antigens encoded by tumor-specific mutated genes, have a key role in therapeutic vaccination. Recent efforts in acquiring omics data through NGS have allowed for the systematic discovery of tumor neoantigens that arise from somatic mutations and are therefore, tumor-specific124. This specificity allows for diverse tumor neoepitopes (peptides that arise from somatic mutations and are recognized as different from self125) between individuals. Identifying these candidate tumor neoantigens on a per-patient basis has led to the development of personalized cancer vaccines124. RNA-Seq data from thousands of samples across 18 different solid tumors from The Cancer Genome Atlas demonstrated a positive correlation between the number of neoantigens per tumor type and T-cell cytolytic activity specific for those tumors124. Furthermore, whole-exome sequencing analysis of 629 colorectal cancers showed that high neoantigen loads are associated with improved patient survival due to the ability to target multiple neoantigens at one time126. Preclinical experiments in both a melanoma model and a transplantable colon cancer model revealed that neoantigen vaccination elicited a selective T-cell response and effectively mediated antitumor activity. In a cholangiocarcinoma patient, adoptive transfer of neoantigen-specific CD4+ T-cells mediated tumor regression, demonstrating the clinical success of this therapy127. Therefore, the concept of targeting multiple neoantigens as a personalized cancer vaccine strategy has been realized and is being further researched and developed.
There is currently only one FDA-approved cancer vaccine, sipuleucel-T, which is indicated for metastatic prostate cancer that no longer responds to hormonal therapy128,129. It is based on the use of dendritic cells taken from the patients blood. In the lab, the dendritic cells are treated with prostatic acid phosphatase (PAP), an antigen that is found on most prostate cancer cells. Antigen-presentation is enhanced, so when the dendritic cells are infused back into the patient, their T-cells react by killing PAP-expressing tumor cells.
CAR T-cell therapies
Genetically engineered CAR T-cell therapies have also shown great promise in the advancement of individualized cancer immunotherapies130. Autologous T-cells are engineered to express a CAR that specifically targets and kills malignant cells or can be directed to remodel the tumor microenvironment through release of soluble factors130. Through recent advances in NGSTs, treatments that target tumor niches with a high degree of specificity can be adapted to account for the tumor microenvironments heterogeneity and complexity. Gathering large data sets that describe different tumor phenotypes/genotypes provides the possibility of precise, individualized design, and optimization of CAR T-cell-based therapies131. Current advancements in genome editing, including CRISPR and gene transfer, have improved CAR T-cell therapy development by increasing their tumor-specificity130.
CAR T-cell therapies exemplify a personalized approach to cancer therapy because they directly prime a patients cells to better combat their own cancer. Thus far, this has been most successful in patients with relapsed or refractory malignancies who are resistant to treatment, particularly in chronic lymphocytic leukemia (CLL), which remains incurable through conventional therapies132. Results from initial trials using CAR-modified T-cells to treat 14 patients with CLL showed 8 out of 14 (57%) successful responses with 4 complete remissions and 4 partial remissions with no relapses. Other successful preclinical and clinical trial data have led to the two first FDA-approved genetically engineered cell therapies. Both are CAR T-cell products, Kymriah and Yescarta, which treat patients with relapsed or refractory B-cell acute lymphoblastic leukemia (ALL) and nonresponsive B-cell lymphoma, respectively. The safety and efficacy of Kymriah was demonstrated in 63 pediatric and young adult patients with ALL with overall remission rate within three months being 83%133. Unfortunately, like with many biologics and gene therapies, Kymriah has proven to show variability in manufacturing, limiting its market availability134. However, with continued advancements in these cell therapy technologies, the ability to tailor each individual patients treatment for their particular cancer is an attainable goal in the near future.
Companion diagnostics
CDx are medical devices that aid doctors in prescribing the most effective, personalized treatments for their patients18. Relevant genetic information for characterizing cancers is found in defined stretches of DNA (i.e., oncogenes). In order to avoid sequencing the entire genome and obtaining extraneous information, some CDx are based off these specific oncogenes and can be used to determine whether or not a person will respond to a certain treatment. Each CDx is associated with a particular drug therapeutic, which, in turn, is associated with a specific genetic abnormality for which it is most effective18.
Gaining insight into the molecular makeup of each patients cancer eliminates the misuse of ineffective and potentially harmful drugs. Studies on cancer and tumor heterogeneity have led to the discovery of various genetic mutations known to drive cancer progression, for example, HER2 mutations in the case of some breast cancers135137. This discovery led to the development of therapeutics to target these precise mutations such as trastuzumab (Herceptin), which is the first approved precision therapeutic to combat breast cancer caused by overexpression of the HER2 gene136,138,139.
A variety of diagnostic methods exist within the category of CDx products, each serving a specific functionality. These include immunohistochemistry (IHC), fluorescent in situ hybridization (FISH), and RT-qPCR ()140. is a current overview from the FDA.gov website of the existing FDA approved CDx devices used in oncology. Many companies have developed different CDx devices specifically for trastuzumab, as this drug has been approved by the FDA since 1998136.
Table 5
FDA-approved CDx for cancer treatments, by company. The FDA is responsible for evaluating the safety and efficacy of all medical devices, pharmaceutical products, and biological products sold in the United States (see ). This table lists FDA-approved CDx for cancer treatment. Each of these products is used to detect a particular omics feature that is linked to a specific cancer phenotype. Positive results from these diagnostic tools help to indicate the potential efficacy of a PPM treatment.
Device manufacturer | CDx name | Drug | Type | Disease | Device/Test specifics |
---|---|---|---|---|---|
Abbott Molecular | VYSIS ALK Break Apart FISH Probe Kit | Crizotinib | FISH | NSCLC | Detect rearrangements in the ALK gene in fixed NSCLC tissue from patients with NSCLC |
Abbott Molecular | PATHVYSION HER-2 DNA Probe Kit | Trastuzumab | FISH | Breast cancer | Detect amplification of HER2/NEU gene in fixed, breast cancer tissue samples. Aid in predicting disease-free and overall survival in patients with stage II, node positive breast cancer treated with adjuvant cyclophosphamide, doxorubicin, and 5-fluorouracil (CAF) chemotherapy |
Abbott Molecular | Abbott Real Time IDH2 | Enasidenib | PCR | AML | Detects single nucleotide variants coding nine IDH2 mutation in samples extracted from patients blood or bone marrow |
Abbott Molecular | VYSIS CLL FISH Probe Kit | Venetoclax | FISH | CLL | Detect deletion of the LSI TP53 probe target from peripheral blood samples from patient with B-cell CLL |
ARUP Labs | PDGFRB FISH | Imatinib mesylate | FISH | MDS/MPD | Qualitative detection of PDGFRB gene rearrangement from fresh bone marrow samples of MDS/MPD patients |
ARUP Labs | KIT D816V Mutation Detection | Imatinib mesylate | PCR | ASM | Qualitatively determines the mutation level of the KIT D816V gene via fresh bone marrow samples of ASM patients |
BioGenex Labs | INSITE HER-2/NEU Kit | Trastuzumab | IHC | Breast cancer | Semiquantitatively determine the overexpression of HER-2/ Neu of fixed normal and neoplastic breast cancer tissue sections |
bioMrieux | THxID BRAF Kit | Trametinib and dabrafenib | PCR | Melanoma | Detection of either BRAF V600E or BRAF V600K mutations in DNA samples extracted from fixed, melanoma tissue. Patients who carry V600E mutations are eligible for dabrafenib and those who carry V600K mutations are eligible for trametinib |
Dako Denmark | HERCEPTEST | Trastuzumab, pertuzumab, and ado-trastuzumab emtansine | IHC | Breast and gastric cancer | Determine HER2 protein overexpression in fixed breast cancer, metastatic gastric, or gastroesophageal junction adenocarcinoma tissues |
Dako Denmark | HER2 FISH PharmDx Kit | Trastuzumab, pertuzumab, and ado-trastuzumab emtansine | FISH | Breast and Gastric cancer | Quantitatively determine HER2 gene overexpression in fixed breast, metastatic gastric, or gastroesophageal junction adenocarcinoma tissues |
Dako Denmark | HER2 CISH PharmDx Kit | Trastuzumab | FISH | Breast cancer | Quantitatively determine HER2 gene status of fixed, breast cancer tissue specimens |
Dako North America | DAKO EGFR PharmDx Kit | Erbitux and vectibix | IHC | Colorectal cancer | Identify EGFR expression in both fixed, normal and neoplastic tissue samples from patient |
Dako North America | DAKO C-Kit PharmDx | Imatinib mesylate | IHC | GIST | Qualitative measure to identify c-kit protein/CD 117 antigen expression in both fixed normal and neoplastic tissue samples |
Dako North America | PD-L1 IHC 22C3 pharmDX | Pembrolizumab | IHC | NSCLC | Using EnVision FLEX visualization system to detect PD-L1 protein in fixed, NSCLC samples |
Foundation Medicine | FoundationOne CDxa | Numerous | PCR | Numerous | Detects: substitutions, insertions, deletions and copy number alterations in 324 genes, select gene rearrangements, genomic signatures such as microsatellite instability and tumor mutational burden, from patient tissue biopsies |
Foundation Medicine | FoundationFocus CDxBRCA Assay | Rucaparib | PCR | Ovarian cancer | NGS-based detection of BRCA1 and BRCA2 (BRCA1/2) alterations from fixed, ovarian tissue samples |
Illumina Inc. | Praxis Extended RAS Panel | Panitumumab | PCR | Colorectal cancer | Detects 56 mutations in RAS genes from DNA extracted from patient tissue samples |
Invivoscribe | LeukoStrat CDx FLT3 Mutation Assay | Midostaurin | PCR | AML | Detects internal tandem mutations and the tyrosine kinase domain mutations D835 and I836 in FLT3 gene from mononuclear cell DNA of AML patients |
Leica Biosystems | Bond Oracle HER2 IHC System | Trastuzumab | IHC | Breast cancer | Semi-Quantitative assay to determine HER2 protein levels of fixed, breast cancer tissues using the bond-max slide staining instrument |
Life Technologies | Oncomine Dx Target Test | Dabrafenib, trametinib, crizotinib, and gefitinib | PCR | NSCLC | Detects single nucleotide variants and deletions in 23 genes from DNA and fusions in ROS1 from RNA, isolated from patient tumor tissue samples |
Life Technologies | SPOT-LIGHT HER2 CISH Kit | Trastuzumab | FISH | Breast cancer | Quantitatively determine HER2 gene overexpression from fixed breast carcinoma tissues using CISH and brightfield microscopy |
MolecularMD Corporation | MolecularMD MRDx BCR-ABL Test | Nilotinib | PCR | CML | Detection of BCR-ABL1 transcripts and the ABL1 endogenous control mRNA in patient blood samples whom are receiving treatment for tyrosine kinase inhibitors |
Myriad Genetic Labs | BRACAnalysis CDx | Olaparib | PCR | Ovarian cancer | Detection and classification of DNA variants in the protein coding regions and intron/exon boundaries of BRCA1/2 genes using whole blood samples from patients |
QIAGEN Manchester | Therascreen EGFR RGQ PCR Kit | Afatinib | PCR | NSCLC | Detection of exon 19 deletions and exon 21 (L858R) substitution mutations of EGFR gene from fixed, NSCLC tissue |
QIAGEN Manchester | Therascreen KRAS RGQ PCR Kit | Cetuximab and panitumumab | PCR | Colorectal cancer | Detection of seven somatic mutations in codons 12 and 13 of the KRAS gene in fixed, colorectal cancer tissue. Treatment of erbitux and vectibix is issued upon a NO mutation test result |
QIAGEN Manchester | Therascreen EGFR RGQ PCR Kit | Gefitinib | PCR | NSCLC | Detection of exon 19 deletions and exon 21 (L858R) substitution mutations of EGFR gene from fixed, NSCLC tissue |
Roche Molecular Systems | The COBAS KRAS Mutation Test | Cetuximab and panitumumab | PCR | Colorectal cancer | Detection of seven somatic mutations in codons 12 and 13 of the KRAS gene in fixed, colorectal cancer tissue. Treatment of erbitux and vectibix is issued upon a NO mutation test result |
Roche Molecular Systems | COBAS EGFR Mutation Test | Erlotinib | PCR | NSCLC | Detect deletion of exon 19 and substitution mutations of exon 21 (L858R) of EGFR gene in DNA from fixed NSCLC tissue |
Roche Molecular Systems | COBAS EGFR Mutation Test v2 | Erlotinib | PCR | NSCLC | Qualitative detection of defined mutations of the EGFR gene in NSCLC patients. Test can be run using fixed NSCLC tissue samples or circulating free tumor DNA |
Roche Molecular Systems | COBAS EGFR Mutation Test v2 | Osimertinib | PCR | NSCLC | Detect T790M mutation of EGFR gene in DNA of fixed NSCLC tissue or ctDNA from NSCLC patients |
Roche Molecular Systems | COBAS 4800 BRAF V600 Mutation Test | Vemurafenib | PCR | Melanoma | Qualitative detection of BRAF V600E mutation in DNA extracted from fixed melanoma tissue from patient |
Ventana Medical Systems | VENTANA ALK (D5F5) CDx Assay | Crizotinib | IHC | NSCLC | Intended for the detection of ALK in fixed NSCLC tissue stained with a BenchMark XT instrument |
Ventana Medical Systems | INFORM HER-2/NEU | Trastuzumab | FISH | Breast cancer | Determines the qualitative presence of HER2/NEU gene amplification from fixed, breast cancer tissue samples |
Ventana Medical Systems | INFORM HER2 DUAL ISH DNA Probe Cocktail | Trastuzumab | FISH | Breast cancer | Determine HER2 gene status via enumeration of the ratio of the HER2 gene to chromosome 17 using fixed, breast cancer tissue from patient |
Ventana Medical Systems | PATHWAY ANTI-HER-2/NEU (4B5) Rabbit Monoclonal Primary Antibody | Trastuzumab | IHC | Breast cancer | Semiquantitative detection of c-erbB-2 antigen (HER2) in fixed, breast cancer tissue specimens using the Ventana automated IHC slide staining device |
Ventana Medical Systems | PD-L1 | Atezolizumab | IHC | Urothelial carcinoma and NSCLC | Assess PD-L1 protein expression levels in fixed, patient tissue samples (stained with OptiView DAB IHC Detection Kit and OptiView Amplification Kit on a VENTANA BenchMark ULTRA instrument) |
It is interesting to note that not all CDx play the role of identifying patients that would benefit from a given therapy, as in the case of the FDA-approved CDx QIAGEN Therascreen141. This RT-qPCR type diagnostic is used to eliminate patients from receiving the drugs Vectibix and Erbitux for metastatic colorectal cancer. The Therascreen PCR kit is meant to detect seven different mutations in the KRAS gene. When patients suffer from a highly mutated form of colorectal cancer, they will no longer benefit from taking Vectibix or Erbitux. Therefore, the doctor will not prescribe them to these patients, preventing the use of unnecessary and ineffective medications that come with negative side effects.
Regulations for PPM
As the technological race advances and new tests and treatments that target specific patient populations are developed, regulatory agencies must devise novel approaches to ensure the safety, efficacy, and security of these products while allowing for innovation142,143. The regulatory landscape has been changing quickly, due in part by the enactment of the Precision Medicine Initiative in 2015, which required the FDA to develop a new platform to evaluate new PPM diagnostics and therapies19, and the 21st Century Act (Cures Act) in 2016, which accelerated medical product development by incorporating the patients perspective and also modernized clinical trial design144. The resulting evolution of the regulatory paradigm has driven an increase in the number of FDA approved PPM products and services. In 2005, only 5% of new drug approvals were PPMs, however, in 2017, over 30% of new drug approvals (16 new therapies) were PPMs9,145,146. The development of regulations that have allowed PPM to enter the market has involved several different agencies, guidance documents, and approaches ().
Regulatory landscape for PPM products and services. A look at the structure of the agencies responsible for regulating PPM products. The FDA is responsible for evaluating the safety and efficacy of all medical devices, pharmaceutical products, and biological products sold in the United States. Most CDx tests and treatment products fall under FDA jurisdiction. The CMS oversees all clinical laboratories in the United States, certifying that they meet quality and proficiency standards for collecting and interpreting clinical data. Generally, the CMS is responsible for approving laboratory-developed diagnostic tests.
Regulatory agencies overseeing PPM products and services
The FDA and the Center for Medicare and Medicaid Services (CMS), both falling under the Department of Health and Human Services (HHS), are the two agencies that hold primary responsibility for overseeing PPM services and products used in clinics, laboratories, and hospitals around the country147.
All medical devices, pharmaceutical products, and biological products sold in the United States are evaluated by the FDA for safety and efficacy before entrance to market, using a risk-based approach142. Different centers within the FDA regulate different types of medical products147, as depicted in . These centers are involved with the approval and oversight of all products that fall into their defi ning categories, and therefore, also oversee relevant PPM products.
Regulations that fall outside of the FDAs jurisdiction belong to CMS, CMS-approved third-party organizations, and state programs, which oversee rules pertaining to all clinical laboratories in the United States through the Clinical Laboratory Improvement Amendments (CLIA)148. CMS certifies that labs meet and maintain certain standards before performing tests and interpreting results on human samples. CLIA requirements generally include qualifications for laboratory personnel, quality systems for lab testing, oversight of test requests and reports, and proficiency testing147.
Types of PPM and associated regulations
A wealth of products, innovations, and tests fall under the umbrella of PPM, and therefore, regulatory agencies must consider the appropriate requirements suited for each type. In this review, the discussion will be limited to CDx, NGS-based diagnostic tests, and laboratory developed tests (LDTs).
CDx regulations.
As defined by the FDA, a CDx is a medical device, often an in vitro device, which provides information that is essential for the safe and effective use of a corresponding drug or biological product149. CDx assist healthcare providers in determining if a products benefits outweigh its risks for patients.
Since CDx are recognized as medical devices by the FDA, they are subjected to the premarket review process. The FDA recommends that a therapeutic product and its accompanying diagnostic test be developed and submitted for approval at the same time; if not, there is a risk of delaying the introduction of the product to the market and limiting access to patients. For example, Herceptin and HercepTest, the first therapeutic product and CDx combination cleared by the FDA, were approved 6 months apart. Although this time gap was relatively short, it was recognized as a potential future risk for products if not developed together9. As a result, the FDA has since released two guidance documents: a final guidance in 2014 titled In Vitro Companion Diagnostic Devices Guidance, which helped clarify its method for conducting simultaneous reviews of a therapeutic product and its associated CDx; and a draft guidance in 2016 titled Principles for Codevelopment of an In Vitro Companion Diagnostic Device with a Therapeutic Product, which explained how therapeutic and diagnostic partners should interact with the FDA when codeveloping combination products. In addition, the FDA has recognized that routine biomarker testing prior to prescribing certain drugs is a class of CDx that will continue to grow. The FDA has therefore begun compiling a table of genomic biomarkers that they consider valid in guiding the clinical use of approved drugs150.
In vitro diagnostics regulations for next-generation sequencing tests and laboratory-developed tests.
The FDA defines an in vitro diagnostic (IVD) as a test to identify patients who are likely to benefit from specific treatments or therapies151. IVDs may be marketed in one of two ways: as IVD kits or as LDTs, which present another set of challenges for regulatory agencies. The main difference between IVD kits and LDTs is that IVD kits are developed by a conventional device manufacturer and are commercially available for healthcare providers, while LDTs are designed, manufactured, and used by a single laboratory147. As a consequence of this distinction, the regulatory jurisdiction of IVD kits and LDTs has generally fallen into two separate agencies, the FDA and CMS, respectively. In addition, if an FDA-approved IVD kit is modified by a clinical laboratory, it will be classified as an LDT (falling into CMS jurisdiction) and will not be required to undergo premarket review; however, if the same IVD kit is modified by a device manufacturer, it will be subjected to the premarket review process (FDA jurisdiction)152. This dichotomy has created more confusion about the proper regulatory path for new PPM products. The approaches that the FDA has taken to ensure the safety and reliability of IVDs are described below.
One type of IVD is NGS-based tests, which are used to find genetic variants that help diagnose, treat, and understand more about human disease151. The thorough sequencing capabilities of NGSTs present a challenge for the current regulatory approaches, which were developed for conventional diagnostics that detect a single disease or condition. In contrast, a single NGS test can yield the equivalent amount of information that millions of traditional tests provide143. Therefore, NGS test development and regulation of NGS-based IVD will require more flexible oversight, which the FDA has pursued by using consensus standards, crowd-sourced data, and open-source computing technology approaches143. According to the FDA, this strategy will enable innovation in testing and research, and will expedite access to accurate, reliable genetic tests151.
In an effort to streamline the regulatory oversight of NGS-based tests by leveraging crowd-sourced data and consensus standards, the FDA released two final guidance documents in 2018: Use of Public Human Genetic Variant Databases to Support Clinical Validity for Genetic and Genomic-Based In Vitro Diagnostics, which describes the process of developing and using FDA-recognized public genome databases to support the clinical validity of a test, and Considerations for Design, Development, and Analytical Validation of Next Generation Sequencing (NGS) Based In Vitro Diagnostics (IVDs) Intended to Aid in the Diagnosis of Suspected Germline Diseases, which provides recommendations for designing, developing and validating NGS-based tests143. In addition, it encourages the development of NGS-related standards by community engagement and standards-developing organizations. Furthermore, the FDA has developed a bioinformatics platform named precisionFDA. This is an open-source cloud-based community that allows individuals and organizations in the genomic field across the world to share data and tools to test, pilot, and validate bioinformatics approaches143,153. This platform further enhances the widespread collaboration that is needed for the technological development of NGS-based tests and demonstrates the FDAs support of this notion as they work to create suitable regulations.
LDTs, which are diagnostic tests that are designed, manufactured, and used within a single laboratory, also fall under the broad category of IVDs147,154. Since these tests are made for in-house use and are not commercially distributed, their regulatory oversight has generally fallen under CMS jurisdiction, which subjects them to CLIA rules. Although the FDA has claimed authority to regulate LDTs, it has generally chosen not to actively exert this power under the enforcement discretion policy147. This policy has been historically applied to simple LDTs, such as in-house vitamin D or sodium assays; however, LDTs have since become more complex and therefore pose higher risks for the patient risks that are similar to those associated with other IVDs regulated by the FDA154. This change in the nature of LDTs, with specific regards to PPM, has led the FDA to occasionally exert its power. This confusion and current lack of a regulatory path for LDTs has made it unclear in which specific cases FDA requirements also apply in addition to those from CMS. For example, in 2005 the FDA subjected the MammaPrint (Agendia BV) breast cancer recurrence assay to premarket approval. The lack of data showing clinical benefits to patients was a major concern for the overseeing FDA officials. Several years later, in 2008, MammaPrint finally received FDA approval, when the markers proved clinical benefits for patients with breast cancer. The MammaPrint assay was reclassified as an in vitro diagnostic multivariate index assay (IVDMIA), which is a type of LDT147. As seen by this example, the FDA has exercised regulatory authority over LDTs to varying degrees under different circumstances. This, in combination with several other factors (e.g., FDA vs. CMS oversight, categorization of IVDs as medical devices vs. LDTs, and different guidance documents/standards applied), has led to confusion and uncertainty in the market, which has hindered biotechnology and pharmaceutical industries investment in the PPM field.
Due to the FDAs evolving concerns regarding the rapid expansion and the intended uses of certain LDTs as CDx for PPM products and services, the agency issued two draft guidance documents in 2014 titled Framework for Regulatory Oversight of Laboratory Developed Tests (LDTs) and FDA Notification and Medical Device Reporting for Laboratory Developed Tests (LDTs). The goal of these documents was to provide clarity regarding the extent of FDA oversight of LDTs. However, after engaging with multiple stakeholders and revising more than 300 comment sets and alternative proposals, the agency recently announced that it will not yet issue final guidance documents on this topic154. This decision was made to allow for further public discussion, and hopefully consensus, on an appropriate regulatory approach. Instead, the FDA published a discussion paper in 2017 summarizing the feedback received and alternative proposals to further advance public discussion on LDT oversight152.
Future regulatory landscape for PPM
Despite the regulatory challenges that exist, the processes outlined by several guidance documents () reflect the FDAs willingness to adapt to the changing landscape of medicine9, along with consideration of feedback from scientists, clinicians, and patients. In response to the increase in the number of PPM products and services, the growing demand for regulatory clarity, and the enactment of the Precision Medicine Initiative and the Cures Act, the FDA began working on the PPM platform over a decade ago. Its aim is to provide a rapidly evolving strategy to approve new PPM diagnostics and drugs, while maintaining high standards of safety and efficacy. Nevertheless, the regulatory landscape of the PPM field is still emerging and is still convoluted due to the complex nature of many PPM products and services that fall under the oversight of multiple regulatory centers. Moreover, the vast data sets that are generated from some PPM products, particularly NGS-based tests, present large challenges for regulatory agencies, as privacy concerns must also be considered. As PPM becomes an even larger part of modern medicine, it is pertinent for discussions regarding regulations to be on-going and for regulatory documents to be continually adapted and updated. Based on recent changes to how the FDA will be changing regulations governing gene therapy in order to streamline review, the agency recognizes the need for these adaptations155. Efforts that address difficult regulatory decisions regarding PPM may begin to cover other controversial topics surrounding this field, particularly in regards to economics and ethics.
Table 6
FDA policy and guidance documents on PPM. A summary of guidance documents developed by the FDA related to PPM regulation and oversight. Regulatory processes for PPM products, which often encompass multiple FDA categories, are complex, but the FDA has been willing to adapt to the continual evolution of PPM treatments as evidenced by the publication of these standards.
Year | Guidance document | Status |
---|---|---|
2005 | Pharmacogenomic Data Submissions | Final guidancea |
2007 | Pharmacogenomic Tests and Genetic Tests for Heritable Markers | Final guidance |
2007 | In Vitro Diagnostic Multivariate Index Assays | Draft guidance |
2008 | E15 Definitions for Genomic Biomarkers, Pharmacogenomics, Pharmacogenetics, Genomic Data, and Sample Coding Categories | Final guidance |
2011 | E16 Guidance on Biomarkers Related to Drug or Biotechnology Product Development: Context, Structure, and Format of Qualifications Submissions | Final guidance |
2012 | Enrichment Strategies for Clinical Trials to Support Approval of Human Drugs and Biological Products | Draft guidance |
2013 | Clinical Pharmacogenomics: Premarket Evaluation in Early-Phase Clinical Studies and Recommendations for Labeling | Final guidance |
2013 | Clinical Pharmacogenomics: Premarket Evaluation in Early-Phase Clinical Studies and Recommendations for Labeling | Final guidance |
2014 | Qualification Process for Drug Development Tools | Final guidance |
2014 | In Vitro Companion Diagnostic Devices | Final guidance |
2014 | Framework for Regulatory Oversight of Laboratory Developed Tests (LDTs) | Draft guidance |
2014 | FDA Notification and Medical Device Reporting for Laboratory Developed Tests (LDTs) | Draft guidance |
2016 | Use of Standards in FDA Regulatory Oversight of Next Generation Sequencing (NGS)-Based In Vitro Diagnostics (IVDs) Used for Diagnosing Germline Diseases | Draft guidance |
2016 | Use of Public Human Genetic Variant Databases to Support Clinical Validity for Next Generation Sequencing (NGS)-Based In Vitro Diagnostics | Draft guidance |
2016 | Principles for Codevelopment of an In Vitro Companion Diagnostic Device with a Therapeutic Product | Draft guidance |
2017 | Discussion Paper on Laboratory Developed Tests (LDTs) | Discussion paper (no enacted guidance) |
2018 | Use of Public Human Genetic Variant Databases to Support Clinical Validity for Genetic and Genomic-Based In Vitro Diagnostics | Final guidance |
2018 | Considerations for Design, Development, and Analytical Validation of Next Generation Sequencing (NGS) Based In Vitro Diagnostics (IVDs) Intended to Aid in the Diagnosis of Suspected Germline Diseases | Final guidance |